This article is part of a special report on the total solar eclipse that will be visible from parts of the U.S., Mexico and Canada on April 8, 2024.
On April 8, 2024, a 115-mile-wide strip of North America will be plunged into darkness. The disk of the moon will slip in front of the sun, obscuring its face and creating a rosy, fluffy crown of flame visible from Mazatlán, Mexico, to Newfoundland, Canada. It will be the last spectacle of its kind for a generation—the next total solar eclipse viewable from across North America will be on August 23, 2044.
Spectators aren't the only ones excited. A solar eclipse is one of the best ways for scientists to study the solar corona, that ring of fire that stands out when the moon blocks our bright star. This feature remains one of the most mysterious parts of the sun. Astronomers originally thought the corona was a feature of the moon—perhaps sunlight reflecting off the lunar atmosphere. But the moon has no atmosphere. It was not until 1806 that Spanish astronomer José Joaquín de Ferrer recognized it was a feature of the sun instead, giving it the name corona, the Spanish word for “crown.” [Related: Here Are the Best Places to View the 2024 Total Solar Eclipse]
On supporting science journalism
If you're enjoying this article, consider supporting our award-winning journalism by subscribing. By purchasing a subscription you are helping to ensure the future of impactful stories about the discoveries and ideas shaping our world today.
We now know that the corona is the sun's shockingly hot outer atmosphere. This atmosphere releases a mysterious “wind” of particles and occasionally unleashes clumps of itself in roiling packages of energy called coronal mass ejections. What we don't know, however, is how or why those things happen.
On April 8, astronomers will train their telescopes on the corona in hopes of demystifying these phenomena. They'll be aided by two new spacecraft that have recently arrived at the sun, gathering data from near and even within the corona. These probes, plus the insights scientists expect to gain from the eclipse, should make this year the most exciting time in solar physics since the dawn of the field.
Solar physics was born during a total solar eclipse in August 1868. Astronomers had just begun using prisms to investigate spectroscopy, splitting the sun's light into its component colors to study the star's chemical makeup. The sun's spectrum contains barcodelike dark lines indicating the presence of elements such as hydrogen, sodium and iron, among others. Two astronomers independently captured the sun's spectrum during the August 1868 eclipse and found that it contained a new line corresponding to a new element—the first element discovered off Earth. They named it helium, after the Greek god Helios, who represented the sun.
The following year, during another total solar eclipse, astronomers in Iowa saw something else odd in the sun's spectrum: a bright green line in the corona that they suspected belonged to a new chemical element. They announced the discovery of coronium, found only in the sun's halo of glorious purple-pink flames. It would be 70 years before another physicist correctly identified coronium as a strange form of iron that had been ionized 13 times, meaning it had half the electrons of a typical iron atom. This state was possible only if the iron atoms had been cooked in a terrific crucible of around two million degrees Fahrenheit. The surface of the sun, however, is 10,000 degrees F. That meant the corona was 200 times hotter than the surface, where the heat and light are emitted. It would be like sitting in front of a campfire in a seat 200 times hotter than the burning wood. Scientists have struggled to explain this immense temperature difference ever since. “That's where modern solar physics really starts,” says Dan Seaton, a solar physicist at the Southwest Research Institute in Boulder, Colo. “Nobody had ever thought that the sun would have had million-degree or hotter plasma in it. What does it mean? What are the consequences of it?”
The biggest consequence of this discovery followed a “trivial calculation,” in the words of Eugene Parker, an astrophysicist at the University of Chicago. In 1958 Parker found that if the corona is two million degrees, the laws of fluid dynamics suggest that it must generate a constant outflow of particles that would eventually travel faster than the speed of sound. Parker's idea was met with resistance, but in 1962 the Mariner II spacecraft confirmed that the particles, called the solar wind, do in fact exist. Scientists still don't fully understand why. They are beginning to get answers, though, thanks to two spacecraft, including one named after Parker.
The Parker Solar Probe, which NASA launched in 2018, is one of the toughest spacecraft ever constructed. Its 4.5-inch-thick carbon-composite sun shield can handle temperatures of nearly 2,500 degrees F and 2.8 million watts of solar energy. Its articulated solar panels can retract behind it for protection, and its onboard water-based cooling system absorbs heat from the solar panels and then radiates it into space. The probe was designed to dip closer to the sun than anything else humans have ever built, sampling its atmosphere, wind, magnetic fields and light.
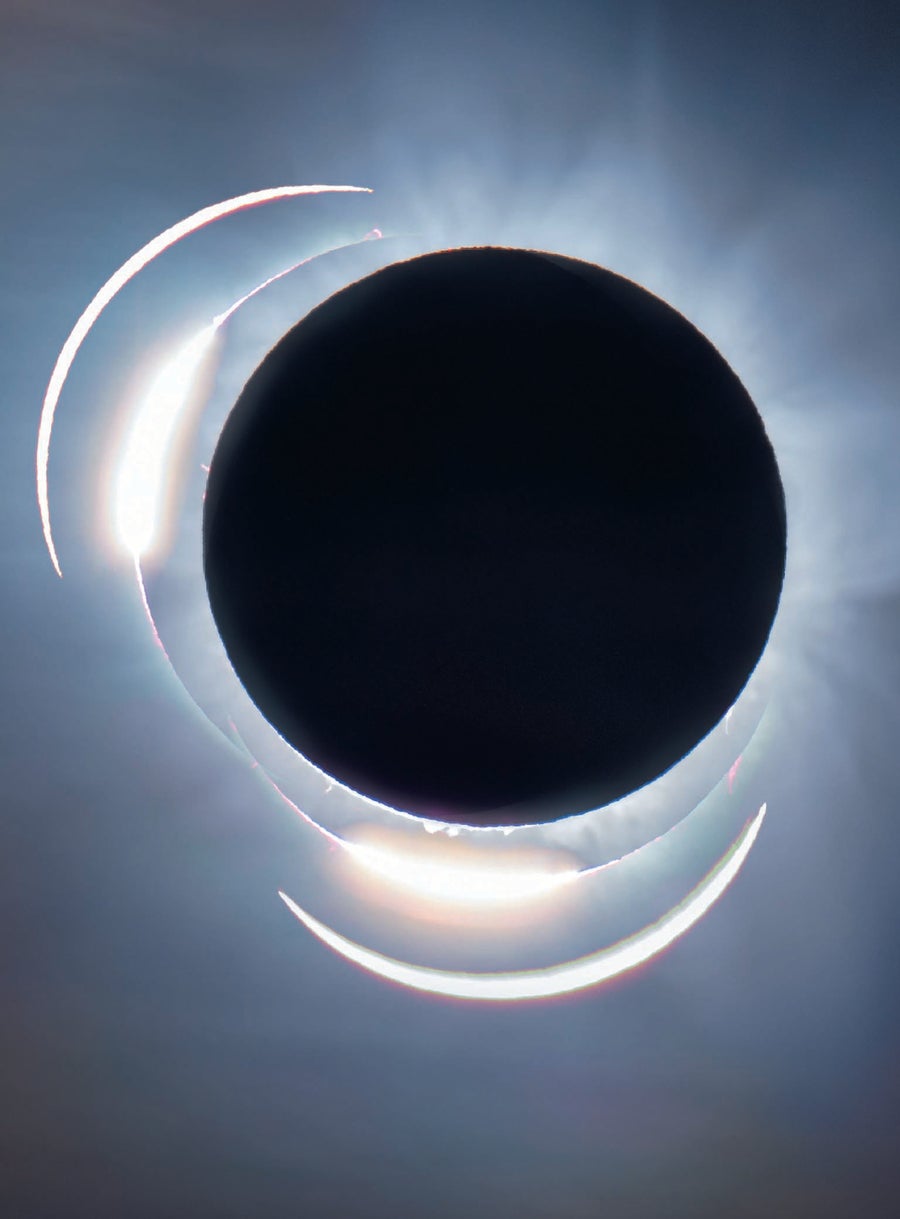
A composite view of the November 2012 total solar eclipse seen from Australia shows totality in the center, along with the “diamond ring” effects created just before and just after the moon completely covers the sun’s face, as well as two thin “crescents” from moments slightly earlier and later. Credit: Alan Dyer
In 2021 the Parker Solar Probe became the first spacecraft to fly through the sun's corona, and since then, it has made nearly 20 close approaches. During its seven-year mission it will complete 24 orbits around our star, using the gravitational field of Venus to slingshot itself ever closer to the sun. Its seventh and final Venus flyby is set for November 2024. Sending the Parker Solar Probe to skim the surface of the sun, diving into the million-degree corona, is a wild, absurdly risky and almost unbelievable thing to try, Seaton says. “It's mind-blowing that the thing works.”
The second craft scientists are counting on is the Solar Orbiter, a European Space Agency probe launched in 2020. It is currently observing the sun from within the orbit of Mercury—not as close as the Parker probe but close enough to study the heliosphere, a bubble of charged particles that the sun blows in all directions and whose edges constitute the end of our solar system. It is the first observatory to make a detailed study of the sun's uncharted polar regions, which are difficult or impossible to see from Earth.
The two spacecraft are the latest in a series of about two dozen sun-observing spacecraft launched since 1961's Explorer 10; of those, 19 are still active, in addition to many solar observatories on Earth. The Parker Solar Probe and the Solar Orbiter will soon be accompanied by other solar-observing spacecraft and sounding rockets, which will observe the sun from Earth's atmosphere. In April 2025 NASA plans to launch the Polarimeter to Unify the Corona and Heliosphere, or PUNCH, which will make three-dimensional observations of the nascent solar wind as it grows and spreads throughout the solar system. Future spacecraft might revisit the sun at higher latitudes, a major challenge for spaceflight engineers but one that would thrill heliophysicists.
“Solar physics really is a very young science,” says Lisa Upton, a solar physicist at Space Systems Research Corporation in Boulder, Colo. “Most of what we know about the sun we have only learned since the dawn of the space age.”
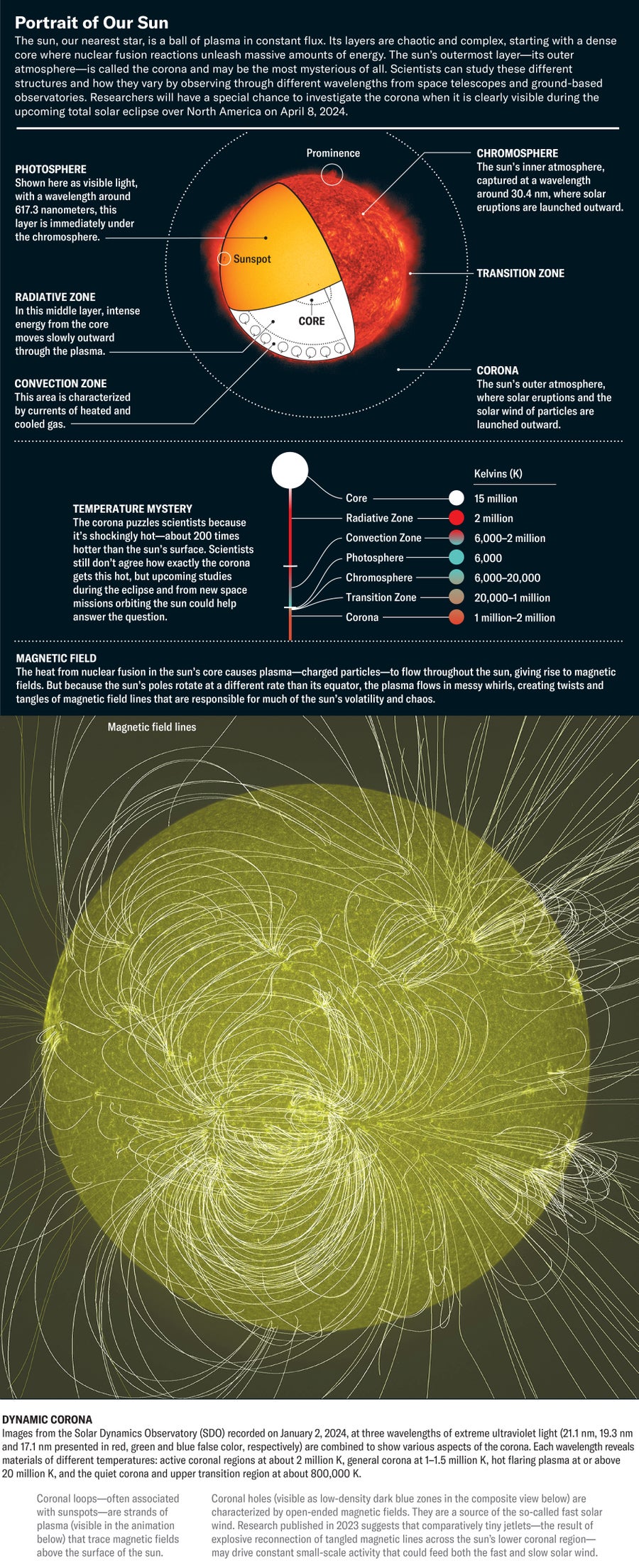
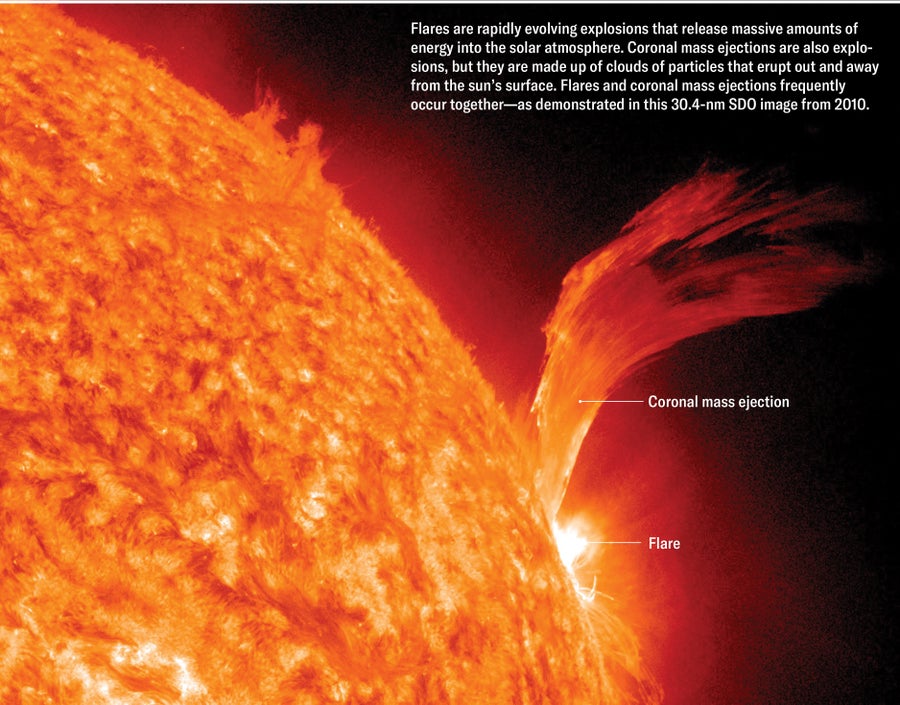
Credit: Jen Christiansen (graphic); NASA/SDO and the AIA, EVE, and HMI science teams (sun imagery); Dan Seaton, Department of Solar and Heliospheric Physics, Southwest Research Institute (consultant)
Space exploration allows scientists, or at least their robotic proxies, to visit the sun up close. The Parker Solar Probe draws so near to the star that researchers have occasionally worried about the spacecraft's health—but so far the probe has weathered whatever the sun has thrown its way. One particularly violent outburst occurred on March 12, 2023, when the Parker Solar Probe was pointing directly at the sun. Sailing just 5.3 million miles from the sun's surface—around a sixth of the distance the scorched planet Mercury reaches in its orbit—the spacecraft's heat shield baked. Sensitive instruments tucked behind it were carefully sampling the sun's outer atmosphere. Then the sun unleashed an unusually fast, abnormally powerful burst of charged plasma. The Parker probe was positioned to fly right through it.
The sun, Earth, the Parker Solar Probe and the Solar Orbiter were all aligned for the coronal mass ejection (CME). More than 40 observatories on Earth were watching at the same time, building an unprecedented view of the event, which rocked the spacecraft like waves tossing a boat. “We were so close to the sun, and it was so intense, we were able to see that in the accelerometer data” that showed the movement and vibrations of the probe, says Jim Kinnison, mission system engineer for Parker Solar Probe at the Johns Hopkins University Applied Physics Laboratory (APL). “I don't think anybody has ever seen that before.”
The CME triggered space weather warnings on Earth because these emissions of charged particles can energize our planet's upper atmosphere, interfering with satellites and radio communications. The Parker probe saw the entire thing—from the CME's generation, to its emission from the sun's surface (or photosphere), to its propagation in the space between our star and our planet. “We thought we kind of understood the structure of these CMEs, but what the Parker Solar Probe showed us with the level of detail we are getting—it is way, way more complex than we thought,” says Nour Raouafi, Parker Solar Probe project scientist at APL. “We came to the conclusion that all the models we have for these events cannot explain everything we are seeing.”
Just like Earth, the sun contains layers. Deep inside is the core, where the sun fuses hydrogen into helium and other heavier elements. Surrounding this orb is the radiative zone, then a convection zone, which generates magnetic fields. The outermost layers are the photosphere (the bright surface), the chromosphere (the transition region) and the corona. These are all dominated by different types of physics, from fluid dynamics and heat flows to magnetic fields and particle acceleration. This complexity makes it very difficult to study the sun as a whole, says Charles Kankelborg, a physicist at Montana State University. “Every now and then,” he says, “I wake up in the morning and think, ‘Wouldn’t it be nice to be an astrophysicist?' Because I wouldn't have all this to work with.”
The new data pouring in mean scientists can finally build a fuller picture of how the sun works. “I've been doing this for more than 20 years, and suddenly it feels like everything is interesting and exciting, and it's all happening at once,” Seaton says. “It's really exciting to come to the office every day, and it wasn't like that [before].”
Scientists think the solar wind originates in the corona, but they're not entirely sure exactly where or how. What's more, the solar wind consists of two types of wind: first the so-called fast solar wind, which can travel at 1.7 million miles per hour and fills the heliosphere, and the slow wind, which streams from equatorial areas at 700,000 miles per hour. (In heliophysics, words like “fast” and “slow” are relative.) The fast and slow winds contain different elements and different numbers of electrons, suggesting they form in different ways. Both are related to magnetic fields.
Those magnetic fields are complex because the sun is a plasma of charged particles. As it burns hydrogen into helium in its core, energy flows to its surface, moving heat through convection. Because the sun rotates at different speeds at its equator and its poles, as magnetic fields rise from its core, they twist and curl amid these solar paroxysms.* Unlike a magnet with a fixed polarity, the sun's magnetic fields are like grains of rice swirling in a pot of boiling water. “The magnetic fields get tangled and twisted and wrapped up into these really complex configurations that aren't intuitive,” Upton says. Magnetic fields with opposite polarities can cancel each other out and make a U-turn, shooting off in a new direction. When this reconnection happens, the new magnetic field lines generate enormous force, like a taut rubber band being snapped, and this force flings plasma out from the sun.
Recent research based on NASA and ESA data found that crossed magnetic field lines sometimes cause certain types of kinks, unleashing S-shaped waves that hurl plasma around. These switchbacks are thought to help generate the slow solar wind. Shortly thereafter, scientists on the Parker team determined how magnetic reconnection may also cause the fast solar wind. Raouafi and his colleagues showed that its flow originates at the base of the corona, from small-scale jets of plasma called jetlets. Later in 2023 solar physicists also found streams of particles that originate in holes in the corona, giving rise to the fast wind. The differences between the slow and fast solar winds may be found in how the magnetic fields are arranged within coronal holes.
If the solar wind is a torrential shower, the jetlets are like the individual droplets that make up the overall stream. The jetlets are found in bright spots where magnetic field lines dive into and spring out from the sun. Taken together, the magnetic reconnection process, coronal holes and jetlets allow the solar wind to rise through the corona and escape the sun's gravity to form the fast solar wind. “These things that we didn't understand about the sun—the extra heating, the way the wind gets up to these high speeds, these weird magnetic switchbacks—these are actually very intimately connected,” says Justin Kasper, a solar physicist at the University of Michigan. “There's kind of a universal picture now that is starting to take shape.”
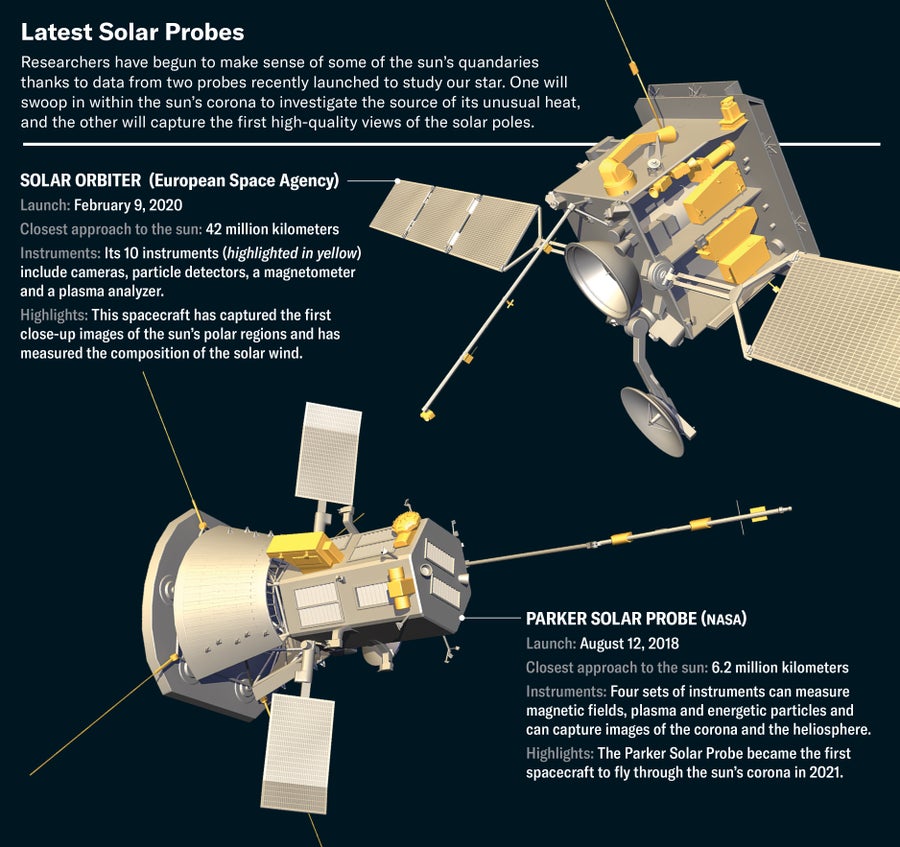
Credit: George Retseck
The sun does not generate its corona through one simple process. Small (again, a relative term) dynamic phenomena are driving larger-scale, obvious phenomena at the sun that we can observe readily but don't understand well, says Craig DeForest, a solar physicist at the Southwest Research Institute. “I think what we're finding is they are all interrelated,” he says. “People have speculated, but the jetlet discovery was the smoking gun showing that these small, explosive events are important to the corona and solar wind.”
Other experts disagree about the jetlets having enough energy to accelerate the solar wind, however; the spurting jetlets might constitute a large part of the fast solar wind, but they might not be what gives rise to it, says Judy Karpen, an astrophysicist at NASA's Goddard Space Flight Center. “But the role of reconnection in all of these seems to be very much the common feature,” she says. The jetlets do seem to have enough energy to keep the solar wind fed, if not to create it, Kankelborg says. The jetlets may also contribute to the corona's extreme heat—another long-standing mystery. Scientists suspect that magnetic reconnection superheats the coronal plasma, and small-scale phenomena such as the jetlets or related phenomena called nano flares might play a role.
The sun is a particle accelerator, a ball of plasma, a self-sustaining thermonuclear reactor, a gale of mass and energy, the source of all life. That we can get close to it—that we're coming closer to understanding it—is a wondrous thing. Studying the sun and its activity connects many disciplines of science, but it also connects us to the other planets. By knowing our mother star, we also come to know about its sister stars throughout the cosmos that are too far away for us to inspect in detail. These studies will even help us understand the planets around those other stars, and they, in turn, might shed light on our sun as well, says C. Alex Young, a heliophysicist at the Goddard Space Flight Center. For instance, scientists hope that their studies of exoplanet systems will help them understand what our sun was like when it was newly born and what it will be like near its death some five billion years from now.
Ultimately, if we can learn the sun's nature, we will come to know ourselves better and understand the physical reasons for an inescapable core fact of our existence: that the sun rises every morning and always will.
*Editor’s Note (4/4/24): This sentence was edited after posting to correct the description of magnetic fields rising from the sun’s core.