From his sniper’s perch on the 32nd floor of the Mandalay Bay hotel in Las Vegas, a lone gunman fired 1,000 bullets from high-powered rifles into a crowd of concertgoers in 2017, murdering 58 innocent people and injuring 869 others. After he committed suicide at the crime scene, the mass murderer’s brain was shipped to Stanford University to seek a possible biological explanation for this depraved incident.
What could the scientists possibly find during such an inspection? Quite a lot, in fact. No genetic test for homicidal behavior is in the offing. But this type of investigation can add insight into how violence is controlled by the brain. Using the same experimental methods that have enabled the tracing of brain circuits responsible for other complex human activities—including walking, speech and reading—neuroscientists now can pinpoint pathways that underlie aggressive behaviors. These new findings help to expose the underlying mechanisms at work in acts of extreme violence, such as the Las Vegas atrocity, but they also help to explain the more commonplace road rage and even a mother’s instantaneous response to any threat to her child.
Physical, sometimes deadly violence is the hub of nature’s survival-of-the fittest struggle, and all animals have evolved specialized neural circuitry to execute—and control—aggressive behavior. In pioneering experiments on cats beginning in the late 1920s, Walter Hess discovered a locus deep within the hypothalamus, a brain area that unleashes violent aggression. It turns out that this is the same spot where other powerful compulsive urges and behaviors are activated, including sex, eating and drinking. When Hess stimulated this knot of neurons using a wire electrode inserted into the brain of a docile cat, the feline instantly launched into a hissing rage, attacking and killing another animal in its cage. The human brain has this same neural structure, labeled the hypothalamic attack area.
On supporting science journalism
If you're enjoying this article, consider supporting our award-winning journalism by subscribing. By purchasing a subscription you are helping to ensure the future of impactful stories about the discoveries and ideas shaping our world today.
This discovery sparked the widely popularized “lizard brain” concept, the assertion that primitive urges in humans spring from an evolutionarily ancient neural core that, in the right circumstance, provokes beastly behavior. Since Hess’s discovery, the vital question confronting scientists for nearly a century has centered on what circuits feed into the brain’s hypothalamic attack region to activate or squelch an attack. Relatively new techniques—optogenetics (an experimental method to switch neural circuits on or off) and fiber-optic cameras threaded into the brains of experimental animals to observe neurons firing during a violent attack—enable some of these questions to be answered. In fact, it is now possible to identify rage and aggression circuits.
For ethical reasons, much of the research tracing the neurocircuitry of violent behavior comes from animal research. Care must be taken in applying terminology used in animal studies to human behaviors and emotions, but clear parallels exist between violence in humans and in other vertebrates. Engaging in physical aggression is potentially life-threatening in any animal, so this behavior is tightly regulated and exhibited only in response to specific types of perceived threats.
Humans and other animals use violent, even deadly aggression instinctively to obtain food, protect their young or defend themselves against bodily injury. But for any of these violent actions—killing prey as opposed to protecting one’s young, for example—separate neural connections come into play.
In addition, many animals are highly social species, and aggression is how social order is established and maintained—picture rams butting heads to determine which one gets to breed with the females. For humans, capital punishment, imprisonment and forced removal of resources (fines and revoking privileges) are all codified forms of aggression to maintain social order. Defending territory, protecting group members and competition are other parallels that enable scientists to extrapolate from studies on experimental animals to find neural circuits in humans for each distinct type of aggression.
From a psychological perspective, human aggression can be sparked by a seemingly endless range of provocations and motives, but from the viewpoint of neuroscience, only a few specific neural circuits in the brain are responsible for this behavior. Identifying them and understanding how they function is still a work in progress, but undertaking this task is critically important. The capability for violent aggression engraved in our brain by eons of tooth-and-nail struggle for survival too often malfunctions in response to disease, drugs or psychiatric impairments and can lead to tragic consequences.
Neural circuits of aggression
The decision to use violent force is fraught with risk, and before a person lashes out, a set of intricate neural circuits extending widely across the brain’s expanse become active. To understand the anatomy of aggression, visualize the human brain as if it had the structure of a mushroom. The thin skin covering the bell of the mushroom equates to the cerebral cortex. A mere three millimeters thick, the cortex is a center of higher cognitive functions—the essence of what makes us human. It is also involved with sensorimotor integration (perception that triggers an action) and even consciousness itself—and it plays a key role in an animal’s deciding whether to exhibit aggressive behavior.
The amygdala, a neural structure located deep underneath the cerebral cortex, which rapidly assesses sensory inputs for possible threats, would be situated at the top of the mushroom stalk, where the rafterlike gills radiate out to support the cap. The amygdala has widely branching ingoing and outgoing links that span from the cerebral cortex to the hypothalamus. The almond-shaped structure acts as a central relay point for sensory information coming into the brain, as well as inputs descending from the cerebral cortex, which convey the results of decision-making and other high-level information processing.
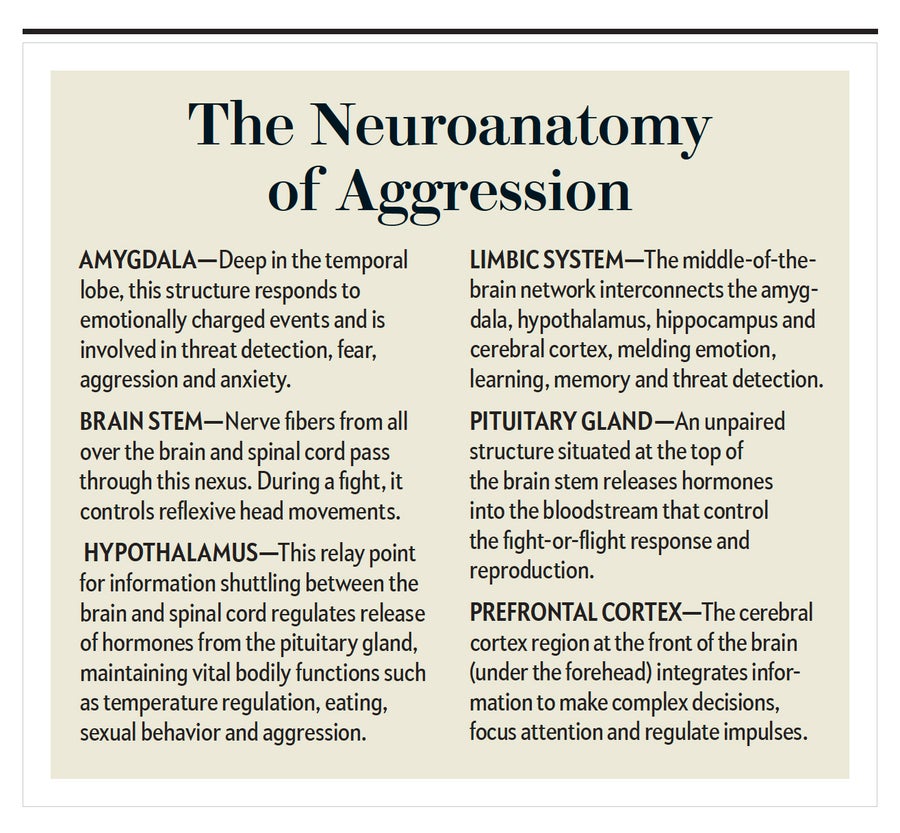
The hypothalamus, also situated at the top of the stalk, is the core brain region that unconsciously controls automatic bodily functions, including heart rate, temperature, breathing, sleep cycles, attention and the release of hormones from the pituitary gland. It is where the emotional drive is generated to initiate an attack. The human brain stem, analogous to the mushroom stalk, is where information is transmitted into and out of the brain through the spinal cord. To depict this analogy accurately, it is important to remember that the human brain is a paired structure, with separate left and right hemispheres. An amygdala, for instance, is found on both the left and right sides of the brain.
Multiple regions controlling aggressive behaviors allow the brain to think fast or slow in response to a threat. The latter more deliberative reaction, however, is the most complex of the two, and the prefrontal cortex is critical for such decision-making. Neuroscientist Simone Motta and colleagues working in the laboratory of Newton Sabino Canteras at the University of São Paulo captured in a 2013 study the biological details of the familiar “momma bear” response, which is by no means solely confined to ursine mothers.
The researchers looked through a microscope at the hypothalamus of a mother rat just after a male intruder entered the cage with the mother and her newborn pups, causing her to attack. After staining the postmortem brain tissue, they identified a protein called Fos in the tiny hypothalamic attack region. Through the microscope, it seemed as if the area had been stippled with a black ink pen. The sudden appearance of Fos, represented by the black staining, resulted from rapid synthesis of the protein as a consequence of neurons in the attack region firing bursts of electrical impulses when the mother was provoked into an assault on the intruder. Other research groups have confirmed a link to aggressive behavior by inserting a fiber-optic camera into the hypothalamic attack region in mice genetically engineered to make neurons emit flashes of light when they fire.
When this cluster of neurons, referred to as the ventral premammillary nucleus, was removed from the hypothalamic attack region before the entry of an intruder, Motta’s group found that a mother was much less likely to respond with a defensive attack. But destroying these neurons did not affect the mother’s responses to a predator cat or other threats. Hess’s electrodes from nearly a century ago were too blunt to reveal fine-level subcircuitry for aggression embedded inside the hypothalamic attack region. New methods of analysis are providing a far more detailed picture.
For this area to be activated by the male intruder, sensory information about the attacker had to be received, processed and relayed through the hypothalamus. All major senses enter the brain via separate neural pathways: visual inputs arrive by way of the optic nerve, the sense of smell via the olfactory nerve. Incoming sensory information reaches the cerebral cortex, where it is analyzed to extract detailed features of a stimulus, and a corresponding signal for each respective sense is sent to another more specialized cortical region. The visual cortex at the back of the head, for example, will extract the shape, color and movement of an object set against the broader visual field and then pass on that information to other cortical regions that bring the perception to our conscious mind—allowing, say, the recognition of a familiar face.
But this complex form of information processing, engaging several different cortical regions in sequence as if building an automobile on an assembly line, takes time. Faced with a sudden threat, a clenched fist thrown toward your chin, the time required to process the visual input and consciously perceive it would be far too slow to dodge the blow. For this reason, a high-speed subcortical pathway that recruits the amygdala has evolved to transmit incoming sensory inputs rapidly to the brain’s threat-detection circuitry. The inflow from the senses reaches the amygdala before it arrives at our cerebral cortex and conscious awareness—the reason why we duck and bat away an errant basketball that suddenly streaks into our visual field and then ask later, “What was that?” The object suddenly intruding into our personal space is perceived as a threat, even though we cannot form an accurate image of it. Similar to a motion detector in a security system, the amygdala has detected an object that should not be there, and it rapidly activates an aggressive response to deal with the threat.
Humans rely heavily on vision, but the sense of smell is more important for many animals. In the Motta experiments, odor most likely alerted the mother rat’s threat-detection mechanism to the male intruder, and this information may have been relayed rapidly to the hypothalamic attack area. Searching the amygdala under a microscope, the scientists saw two spots there that were clearly stained for Fos in response to the intruder’s attack. Both these locations in the amygdala—within the medial amygdala nucleus—receive input from the olfactory region. The premammillary nucleus region of the hypothalamus, where the maternal aggression response is centered, has neurons in it that are known to respond to odors only from the opposite sex.
Another part of the amygdala, the posterior nucleus, also showed ample evidence of Fos staining. Neurons there have hormone detectors (mineralocorticoid receptors) to link stress to a trigger for aggression. In other studies on aggressive male rats, the animals become docile when these receptors are blocked. This observation explains in part how diverse aspects of a given situation, whether stress or other factors, can lower the threshold for inducing aggression.
Human experiments
The intention of any of these studies is to determine whether activating or switching off a particular brain area produces a specific behavior. Animal studies, however, cannot reveal much about what constitutes the actual sensations involved in any of the resulting behaviors. Stimulating the rat brain with an electrode might induce pain that then provokes a violent reaction, giving no hint about whether the reaction resulted directly from the activation of a brain center linked to aggression.
Some experiments, though, have been performed on human subjects, leaving no doubt that the amygdala unleashes intensely violent emotions. In the 1960s, when the late Spanish neuroscientist José Manuel Rodríguez Delgado stimulated an electrode in a woman’s right amygdala as she was peacefully playing guitar, she stopped strumming and singing, threw the instrument away in a fit of rage and started to attack a nearby wall. Such powerful emotions unleashing violent behavior must override competing impulses. The risk of deciding to launch an attack could lead to retaliation that puts the aggressor at risk of severe injury—or death—or otherwise provoke the shame that results after fleeing in fear from a threat.
The neural seats of blind rage in both rats and humans are part of an expansive neural network that reaches beyond the amygdala to unleash violent behavior. Researchers have discovered a locus in the septal region, part of what is called the subcortical limbic system, that switches on after a rat fights off an intruder to protect her young. The septal area drives intense emotional responses, such as explosive rage, and is also active during sex and other rewarding activities. In the 1950s James Olds and Peter Milner showed that rats with electrodes implanted in the septal region would press a bar to deliver an electrical stimulus to neurons there to the point of exhaustion—up to 5,000 times per hour.
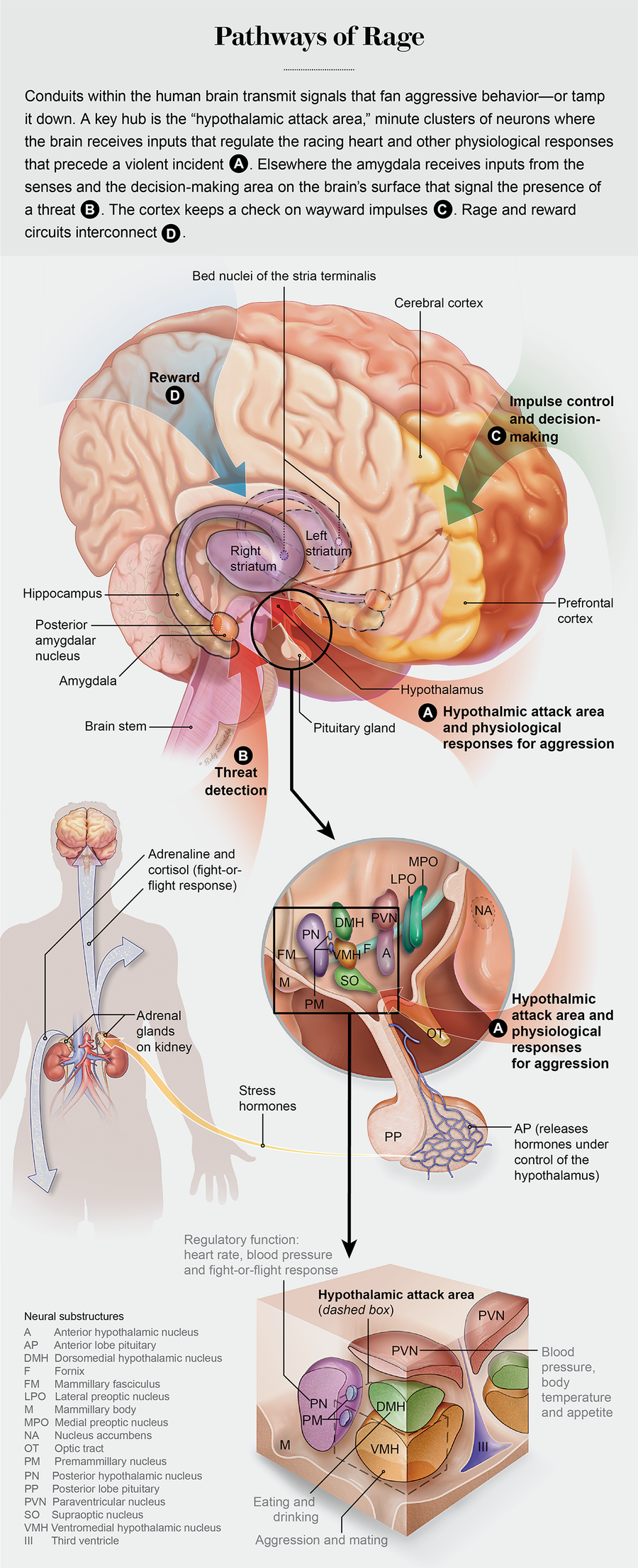
Credit: Body Scientific
A counterpart to these experiments has been performed with human involvement. When Delgado stimulated patients’ septal regions, they were suddenly overcome with strong sexual feelings that ultimately built to an orgasm. One patient became flirtatious and even offered to marry the therapist.
In what are now recognized as unethical studies published in 1972, psychiatrist Robert G. Heath of Tulane University attempted to “cure” a young man of homosexuality. He implanted electrodes into the septal region of the man’s brain to enable the physicians or the patient himself to provide neural stimulation that delivered sexual pleasure while watching heterosexual pornographic films and while having sex with a female prostitute. Heath reported that the subject stimulated himself to the point of euphoria. (His sexual orientation remained unchanged, however.)
Neurons in this part of the septal area (the bed nuclei of the stria terminalis, or BNST), which in animal studies were activated during maternal aggression, also display receptors for norepinephrine, a neurotransmitter involved in stress responses. This brain region connects to the hypothalamus for the control of autonomic responses and release of hormones, such as oxytocin or the neurotransmitter dopamine, that regulate stress, mood and anxiety: it also receives input from the cerebral cortex.
The circuitry of aggression goes both high and low. The prefrontal cortex can inhibit or stimulate the limbic system, squelching an impulse or initiating a violent action based on the deliberation that takes place in high-level cognitive processing areas. This “top-down” control from the prefrontal cortex contrasts with its “bottom-up” counterpart, the rapid, reflexive response to a sudden environmental stimulus, as when the errantly thrown basketball is deflected without any conscious thought. Animals and people with weaker connections from the prefrontal cortex to the limbic system encounter difficulties with impulse control.
The brain’s reward centers, including the striatum and nucleus accumbens, where the neurotransmitter dopamine acts, are another component of the aggression circuitry. Many drugs of abuse and addiction—methamphetamine and cocaine, for example—increase the reward-modulating dopamine to trip this circuitry. When a male rat succeeds in defeating a trespasser entering its cage, the animal will repeatedly activate a lever to open the passageway to admit the intruder to fight it again. If dopamine signaling is blocked with a drug, the male rat will cease to initiate another battle.
The rewarding aspect of aggression, including feelings of superiority and dominance, underlies several forms of this behavior, but in particular, the hedonistic component of bullying, as well as psychopathic and brutal criminal violence. In modern society, where our food needs are supplied by supermarkets, the missing sense of reward that comes from a successful kill can be satisfied through recreational activities such as hunting and fishing.
Sex differences
The single most important factor in predicting aggressive behavior is one’s sex. According to 2018 statistics from the Federal Bureau of Prisons, 93 percent of inmates are male. The association between aggression and being male is prominent in the animal kingdom, demonstrating that the relation between violence and sex has a strong biological basis. Hormonal influences on neural circuits controlling aggressive behavior are a large contributor, but the selective pressure on males, especially in social mammals, including most primates, has promoted attributes that increase the probability of aggressive behavior in the quest to find a mate, achieve elevated social status, acquire food, and defend territory and tribe.
Neuroscientist David Anderson of the California Institute of Technology and his colleagues have investigated the neural circuitry that explains the perplexing association between sex and violence. Their research has uncovered part of the mechanism for how the same brain circuitry could be involved in extreme opposites such as love and hate. From a physiological perspective, several common features tie aggression to mating. Both behaviors evoke intense states of arousal and, when successful, potent feelings of reward. In the natural world, aggression and mating are often interrelated, and both are regulated by similar environmental influences and internal body states. Male animals, for example, are more aggressive during mating season.
It has been known for some time that mating is also controlled by the hypothalamic attack area and that stimulation from electrodes placed there can induce copulation or aggression. Using Fos staining to identify highly active neurons, the researchers found that cells in the hypothalamus became active immediately after mice engaged in either an aggressive encounter or mating. Dayu Lin, while working in Anderson’s lab before becoming a professor at New York University, implanted microelectrodes into the hypothalamus of mice and found that neurons were buzzing during fighting and mating—some individual neurons fired during one behavior and not in the other, but some turned on during both activities. By threading in a fiber-optic strand to shine a laser beam that made genetically modified neurons generate electrical impulses in response to light, Lin and her colleagues spurred the mouse to initiate an attack or copulate. They used the laser to drive neuron firing at different frequencies and switch between behaviors.
Losing it
Using these new findings from the lab to help explain a mass killing is still an aspirational goal. But an incident that occurred more than 50 years ago may have set in motion a process of inquiry that could one day fend off horrific headlines. On August 1, 1966, Charles Whitman, a troubled former U.S. Marine, stabbed and shot his mother to death and killed his wife with a knife in their respective homes before going to a tower on the University of Texas at Austin, campus with a footlocker packed with three knives, 700 rounds of ammunition and seven guns. Whitman killed 14 people from his sniper’s perch and injured more than 30 others. He left a note requesting that his brain be studied after his death to determine if he was mentally ill.
Forensic analysis of the killer’s brain found a small tumor, glioblastoma multiforme, near the amygdala. The team of experts conceded in its written report: “The highly malignant brain tumor conceivably could have contributed to his inability to control his emotions and actions,” but the experts were unable to make a conclusive determination that the cancer had anything to do with Whitman’s mass murders or his apparent mental illness. After all, many people suffer brain injuries and tumors, but they do not become violent killers; Senator Ted Kennedy and Senator John McCain, for example, were both stricken with glioblastoma multiforme.
Thus far no abnormality has been reported in the brain of the Las Vegas mass murderer Stephen Paddock—and one may never be found. If pathology does turn up, it will still be impossible to find a cause-and effect relation between the brain tumor and the heinous crime. Moreover, statistics from the MacArthur Violence Risk Assessment Study indicate that people with mental disorders are no more likely than others to be violent.
The odds are that no neurological abnormality will be found in the brain of the Mandalay Bay sniper. The major risk factors that predict violent behavior are youth, male sex, substance abuse and lower socioeconomic status. One third of self-reported violent acts committed by people without diagnosed mental illness and seven out of 10 violent crimes among the mentally ill are associated with substance abuse, according to a 2003 review by Heather Stuart of Queen’s University in Ontario. Our knowledge of how alcohol or cocaine impairs the brain’s neural circuitry for aggression leaves little doubt about the connection between substance abuse and violence.
New understanding
The committee of experts who examined Whitman’s brain articulated a larger reason for its inability to link the brain tumor to the crime—quite simply, it had to do with the basic lack of a scientific grasp of the brain in 1966. “The application of existing knowledge of organic brain function does not enable us to explain the actions of Whitman on August first,” the report notes. “This case is a dramatic indication of the urgent need for further understanding of brain function related to behavior, and particularly to violent and aggressive behavior.”
The MRI machine did not exist in 1966, and the entire field of neuroscience was in its infancy. More recent research using modern techniques to explore the new neuroscience underlying aggression is now yielding knowledge that might have helped Whitman’s search for closure.
Psychiatrist Bernhard Bogerts of Otto von Guericke University Magdeburg in Germany and his colleagues used MRI and CT scans to examine the brains of violent and nonviolent prisoners. The research found significantly higher incidence of brain abnormalities in violent offenders than in nonviolent ones or a control group. For instance, 42 percent of the 162 violent prisoners had at least one abnormal area versus 26 percent of the 125 nonviolent inmates and 8 percent of the 52 individuals in the control group. The pathology showed up in the prefrontal cortex, the amygdala and other regions responsible for control of the amygdala and hypothalamus.
Information uncovered about the neurocircuits of aggression may provide a path to new answers, but it may also raise fresh questions. Both genes and experience guide the development of neural circuits differently in every individual, an explanation for the varying intensity and types of aggression exhibited in humans or experimental rodents. The prefrontal cortex does not fully develop until the early 20s in humans, pointing to why juveniles should not be held criminally responsible as adults in the U.S.
This sluggish neurodevelopment process provides some degree of biological insight into the seemingly incomprehensible waves of tragic school shootings rocking the country. Ultimately interventions to reduce violent behavior may be possible by regulating neural circuits of aggression with drugs, precision surgery, brain stimulation or other methods.
The emerging evidence of neurological abnormalities in people incarcerated for violent behavior raises ethical questions of legal culpability and whether psychiatric assessment of mental health patients should include EEG and brain-scanning assessments to look for signs of pathology. That may be what Whitman was seeking as he packed his footlocker and wrote out his suicide note asking that his brain be examined after the bloodbath he was about to commit.