Mercedes Diaz tramps into a muddy soybean field and runs her brightly manicured fingers through the limbs of dozens of knee-high plants. As she checks the stems, pods and leaves, she rattles off a list of possible maladies under her breath: pod borers, frogeye leaf spot, white mold. Diaz spots a tangle of mottled leaves and shouts, “SDS!”—signaling sudden death syndrome. She plucks one of the leaves and hands it to me. I turn the crinkly, palm-sized leaf over in my hand. Irregular holes riddle its surface, along with ugly brown spots tinged in yellow—the effects of a toxin produced by the SDS fungus that courses through the plant, robbing it of its pods and chewing up the leaves from the inside out. SDS is one of the top crop killers in the U.S. According to the United Soybean Board, it cost farmers more than 60 million bushels in lost yields in 2014. And yet Diaz could not be happier to see it in her field.
A plant pathologist, Diaz is one of a myriad of researchers hunting for new ways to protect crops from threats and to dramatically boost yields. In 2016 her team coated seeds with thousands of different microbes and planted them, along with control plots of untreated seeds, in half a million spots throughout the Midwest and South. Along the perimeter of these fields, the researchers deposited sentinel plots—sown with varieties susceptible to disease that act like the proverbial canary in the coal mine, warning of harms that might come to the rest of the crops. When Diaz finds SDS or some other blight in the sentinel plots and not in the test plots, it could be a sign that the microbes are working, helping to produce healthier, more abundant crops.
Yet on this rainy September day, Diaz finds that both the test plots and the control plots have escaped the sentinels' fate. The microbes did not make a difference—or did they? Even an improved yield as high as six bushels per acre (over an average of about 50 bushels per acre for soy) is nearly impossible to spot on sight. She will have to wait for the plants to be harvested and the data to be analyzed to find out if any of the microbes helped.
On supporting science journalism
If you're enjoying this article, consider supporting our award-winning journalism by subscribing. By purchasing a subscription you are helping to ensure the future of impactful stories about the discoveries and ideas shaping our world today.
“We need to stop looking for silver bullets,” says Jan Leach, a plant pathologist at Colorado State University. “This is not a problem that any one of us can solve on our own, and it will require very diverse teams of people working together in ways that we've never worked before.” Leach and other scientists are championing a more holistic approach, one that takes into account how all the components on a farm—the plants, soils, microbes, insects and climate, known collectively as the phytobiome—interact to determine crop yields. The concept harks back to the writings of 19th-century naturalists Alfred Russel Wallace and Charles Darwin, who presented nature as a vast, interconnected web where species constantly adapt to the changing environment around them.
Take Diaz's beloved soybeans. When an insect lands on a soybean leaf, the plant might respond by secreting volatile chemicals through its roots, which, in turn, alter the microbial makeup of the soil. These microbes could then switch on an array of genes in neighboring plants, putting them on high alert for a potential attack. Such critical plant defenses are sensitive, however, to various environmental factors and could, for example, be vulnerable to climate change. Pathogens have their own amazing tricks. Some can shoot themselves off the surface of a leaf like a cannonball, catching a ride on air currents from field to field, continent to continent. From the clouds, some strains of high-flying microbes can even influence the weather, summoning rain and hail to return them to the earth.
Scientists have suspected this complexity for centuries, but only recently have advances in technology enabled them to map these intricate interactions in the hope of developing more systematic, sustainable solutions for agriculture. Using gene-sequencing tools, they can now measure all the microbes in the soil, even the rare varieties or persnickety strains that are impossible to grow in the laboratory. They can track how these microbial communities shift in space and time, perhaps as the result of a surge in fertilizer or a drop in temperature. They can record the conversations that microbes, plants and other organisms have with one another and try to decipher how that chemical communication drives crop productivity and health.
One day a farmer may be able to ride a specially equipped tractor into a field and take a comprehensive census of its microbial residents, along with the more typical measurements of “precision agriculture”—such as the soil's moisture levels and nutrient content. Those factors could then be combined with data on previous crop yields, potential pests and pathogens, and projected weather patterns to predict which combination of seeds, nutrients, chemicals and microbes should give the highest yield.
The movement to make this vision a reality has only just begun. In 2016 a diverse band of scientists released an ambitious plan for transforming the future of agriculture, Phytobiomes: A Roadmap for Research and Translation. With the Roadmap came the creation of the Phytobiomes journal, an academic publication, and the Phytobiomes Alliance, an industry-academic collaboration that includes more than a dozen entities—newbies such as BioConsortia and Indigo and familiar names such as Diaz's employer, Monsanto. Over the past couple of years these companies have invested heavily to ramp up research and development, vying for their share of the global agricultural biologicals market, projected to reach $10 billion by 2020.
They see the ground below our feet—and its vast network of resident microorganisms—as vital to that effort. Soil-dwelling bacteria and fungi can help plants grow, cope with stress, bolster immune responses, and ward off pests and diseases. Farmers have known some of this since the late 1800s, when they began treating their pea and bean patches with the bacterium rhizobium, which adds nitrogen to the soil. Nowadays dozens of products based on the soil microbiome are on the market, with more on the way. Monsanto, in partnership with Denmark-based Novozymes, is betting big on microbe-laced seeds developed through the massive experiment that Diaz is part of. Other researchers are trying different tacks, such as altering the genome of crop plants in ways that would attract useful microbes or manipulating pest-plant communication so that crops can better detect and respond to a threat. Given the phytobiome's complexity, the possible avenues of influence are infinite. So are the dead ends. The challenge is to hit pay dirt in time to avert famine.
Notes from underground
An hour outside of St. Louis, the cornfields look withered and pale in the mid-September sunlight. Stretches of soybeans resemble 1970s-style shag carpet dyed in shades of avocado green and harvest gold. I am traveling with Diaz and her colleagues to an unmarked site on the fringes of Stonington, Ill. As I step into a puddle, I grab my copy of the field map showing where thousands of microbe-laden seeds were sown. The microbes had been grown in vats of rich broth, coated on the seeds inside giant stainless-steel bowls and then kept frozen until planting time. Once the seeds sprout, these microbes spring to life, but what happens next is shaped by the multiple factors that make up the phytobiome.
I enter a maze of cornstalks behind Diaz, who shows me several sentinel ears covered in pink mold, swarming with tiny whiteflies. Plants lack a bona fide immune system, but they have evolved a number of maneuvers to fend off insects. Some plants can thicken the walls of their cells so that intruders cannot get through, or they send toxic chemicals out to their roots or leaves to make them less palatable. Nicotine, caffeine and even the tannins that give red wine its astringent quality are all products of plant defenses.
Centuries of breeding and decades of genetic engineering have sought to enhance plant defenses and build other useful traits that increase crop yields. For example, more than half of the corn now grown in the U.S. contains a gene from an insect-killing bacterium called Bacillus thuringiensis, or Bt, that enables the corn to destroy beetle larvae. Now scientists are searching for other, phytobiome-related traits that could help promote plant health. They have discovered that plants spend as much as 30 percent of their energy trying to attract the right microbes and repel the wrong ones. Jeffery Dangl, a plant biologist at the University of North Carolina at Chapel Hill, is investigating ways to alter the plant genome to further cultivate this microbial entourage. He recently discovered a gene that shapes the communities of bacteria living in and around roots to soak up more phosphate—a nutrient in dwindling supply—from the soil.
Other phytobiome research focuses on resistance to bugs. Typically a plant can detect when pests are afoot by the presence of special chemicals called elicitors in the saliva of chewing insects. Gary Felton, an entomologist at Pennsylvania State University, and his colleagues discovered that some beetles and caterpillars can mask these telltale molecules by spitting up gut microbes onto the leaf, tricking the plant into reacting as if it were bathed in bacteria rather than ravaged by bugs. The misguided response to microbes actually disrupts the plant's ability to defend against insects. Recently Felton showed that feeding beetles a particular kind of bacteria skewed their microbiome enough so that they could no longer fool the plant [see box below].
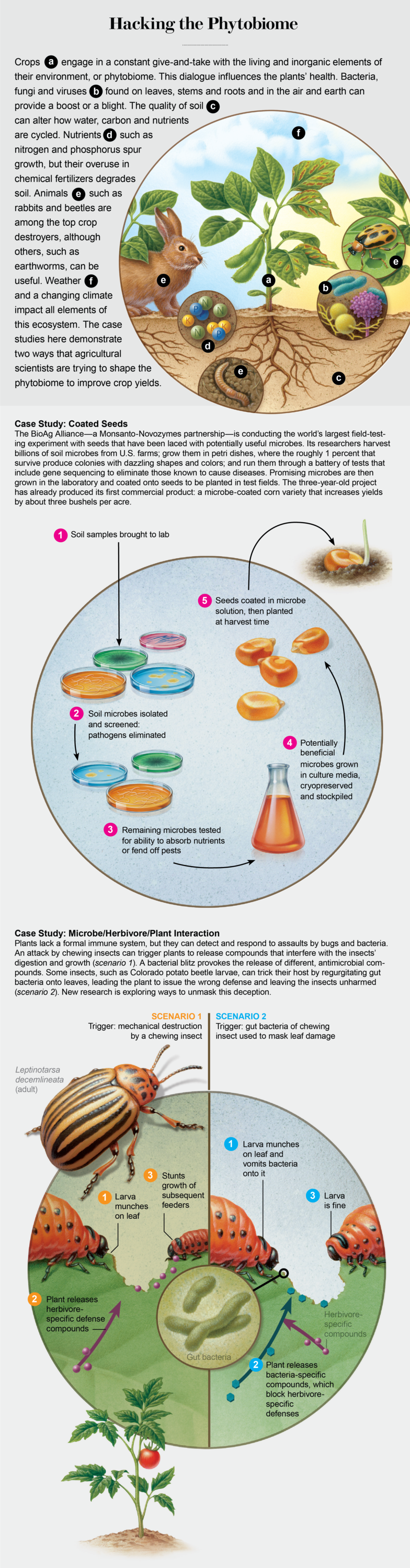
Credit: Cherie Sinnen
The next green revolution could spring from any or all of these methods of shaping the dialogue among plants, pests and soil dwellers. But first, there are experimental crops to tend and massive numbers to crunch.
In the late summer of 2015 a fleet of machines fanned out into fields from Louisiana to Minnesota and North Carolina to Nebraska to harvest each of the test plots of corn and soy planted by the BioAg Alliance, as the Monsanto-Novozymes partnership is known. Data from the burly harvesters are streamed in real time back to Monsanto's data center in St. Louis, as well as to Novozymes's facility in Research Triangle Park, N.C. Scientists in both locations are known to hunker around their computer screens to watch the numbers come in from the fields. “It's like watching a horse race in slow motion,” says Scott Schaecher, biotechnology data strategy lead at Monsanto.
They have found it best not to put too much stock in the early results. The raw data can be deceptive because they do not take into account factors that might give a particular microbe a head start or make it fall behind. The soil microbiome can vary from plot to plot, even across a field. Weather can wreak havoc: seed coatings can be washed off by early rains, and one year the alliance lost thousands of plots to Hurricane Joaquin. In addition to half a million yield points, the team collects 50 different measurements on each of its soil samples. Add in other phytobiome data, and you end up with terabytes of information, what Schaecher calls either “a statistician's carnival or nightmare.”
On his office computer, Schaecher pulls up a U.S. map decorated in red and green dots like a Christmas tree: green for the microbes that boosted yield, red for those that lowered it. They represent 2016 results from five corn fields. He and his team can break down the data according to soil and environmental characteristics, weather, and insect and disease pressure. They can zoom in on the locations with high levels of Diaz's SDS to see if they can identify any microbes that excelled under those conditions.
The team uses a “field first” strategy, which means it skips the typical greenhouse experiments and tests its candidates directly in the field. As a result, the researchers have no idea which, if any, microbes will give an advantage. In 2014, the first year of the field trial, they planted seeds coated with 500 different strains. Ninety percent of the microbes failed. In 2015 they put 2,000 microbes into the race, including the winners from the first year. After that trial, only a handful of the original contenders remained, along with a couple hundred of the newbies. In 2016 the scientists planted another 2,000 strains, made up of the top performers and a batch of new recruits. Three years into the experiment, only a single microbe from the initial round—plus hundreds from later rounds—remained in contention. The team is not looking for one-hit wonders—it wants Triple Crown winners that perform consistently, year after year, on multiple fields.
Risky Business?
Working with all-natural microbes—taken from farmland, grown in the lab and then returned to the farm—might seem like a harmless proposition, free of the kind of controversies associated with genetically modified organisms (GMOs). Nevertheless, it raises a number of concerns. Messing with the microbial milieu could affect the flavor of a crop, much as soil composition influences the taste of wine. A yield-boosting bacterium might possess pathogenic properties that prove harmful to human health. Long-term applications of plant probiotics could change the natural dynamics of soil, fueling the proliferation of some microorganisms while driving others to extinction. There is also a risk that seed coatings, like many agents applied to a field, could slough off one crop and contaminate another.
Schaecher says the BioAg Alliance works hard to avoid such problems. It puts its microbial strains through a battery of tests before introducing them into the field. It sequences each genome to make sure the microbe bears no resemblance to known human pathogens and runs other tests to assess if it might be toxic to the environment or spread to another crop. He and his team consult regularly with the U.S. Department of Agriculture, which decides whether a permit is required before a particular microbial species can undergo field-testing. Organisms with benign duties such as fixing nitrogen or solubilizing phosphate typically get a pass. Those with more dangerous jobs such as killing off other bacteria or fungi require more paperwork.
Among agricultural researchers, the bigger concern is not that newly introduced species will take over or spread to other crops but rather that they will not stick around long enough to do much of anything, says Gwyn Beattie, a plant pathologist at Iowa State University and one of the authors of the Phytobiomes Roadmap. A spoonful of soil contains about 50 billion microbes, a mix of up to 10,000 different species. Researchers can add millions of one strain to the soil and not make a dent. “If you throw one person [at a time] into New York City, the vast majority of people you throw in there do not change New York City,” Beattie says. “It is like that in a microbial community. Introducing organisms rarely has an impact at all, and that's actually the biggest frustration.” (A similar challenge has mired the human probiotics industry, which aims to enhance the multitrillion-member microbial community in the human gut. Its powders, pills and potables have been promoted for treating ailments ranging from diarrhea to depression, but few studies show any measurable effect.)
Still, Monsanto's activities have worried large segments of the public, which have accused the company of endangering human health, trampling the rights of farmers and monopolizing the food supply. Most of this ire arose in the mid-1990s, when the agricultural giant launched a line of GMO crops. Since then, two opposing narratives have emerged: one in which the company develops seeds that double yields and heroically overcome food shortages; the other in which its products defile farmlands and cause cancer. In 2016 the National Academy of Sciences completed what may be the most thorough examination of GMOs to date and found neither to be true. Its report concluded that genetically modified crops were just as safe to eat as conventional crops but that “there is no evidence” that GMOs have boosted progress on yields.
The primary benefit from genetically modified soybean, cotton and maize, the report suggested, was “favorable economic outcomes for producers who have adopted these crops.” When I asked about the academy's lackluster findings, a Monsanto representative admitted that the company had shifted its sales pitch from feeding the world to helping farmers get the best possible yields at the lowest cost. Monsanto's sales totaled $13.5 billion in 2016, nearly $10 billion of which came from seeds, many of them genetically enhanced. The advances of the past two decades have saved money for farmers and made money for industry, but the need for more food remains urgent.
Tomorrow's Harvest
The seeds planted on American farms today are not your grandfather's seeds. They typically carry as many as 14 different genetically engineered traits, piled on top of one another. These “stacks,” as they are called in industry speak, are often accompanied by a host of other potentially yield-boosting products, including fertilizers, herbicides and, more recently, biologicals such as the BioAg Alliance's microbial seed coatings. Yet there is still a lot we do not know about what goes into a healthy crop—and scientists in academia and the government, as well as big agriculture, are all trying to figure it out.
Even with advances in sequencing technology, scientists have identified only 1 percent of the microbial species in soil. The murky nature of soil has made visualizing what is happening underground difficult. Scientists have had to resort to destructive end-point studies—shoveling up scoops of soil and taking a rough survey of its microbial constituents, like a giant running its hands across the earth to capture a sample of humankind. That approach might indicate which people are there but not what they were doing or how they were interacting before their world was turned upside down.
A few years ago a team in Scotland concocted a see-through artificial soil that allows researchers to spy on the microbial communities associated with plant roots. Elizabeth Shank, a microbiologist at Chapel Hill, has been using this transparent soil to study the microbes' signals. These chemical messages range from lethal—70 percent of antibiotics are derived from metabolites that microbes use to off one another—to productive—some microbes use them to announce they are gathering to form a biofilm so that they can stick onto the surface of roots. In November 2016 Shank presented her work at a symposium on phytobiomes in Santa Fe, N.M. She explained that by painting different microbial metabolites with fluorescent markers, she can watch messages travel between communities as they react to events such as the planting of a seed, a rise in temperatures or an invasion by pathogens.
Her innovation may yield big payoffs. Or not. Many academics such as Shank are licensing their discoveries to businesses or launching their own start-ups. The commercial ecosystem is thrumming with new entities, collaborations and mergers, including September 2016's $66-billion purchase of Monsanto by Bayer. The U.S. government has also gotten into the game. The 2014 “farm bill” allotted $200 million to establish the Foundation for Food and Agriculture Research (FFAR) to encourage academic-industry partnerships. In July 2016 FFAR convened a group of experts to brainstorm about how to reap the most benefits from the phytobiome.
Kellye Eversole, executive director of the Phytobiomes Alliance, says that although academia, government and industry might share similar goals, their methods are sometimes at odds. Because companies are beholden to their investors, they can have a short-term, profit-driven mentality. “Not having fundamental research and building that long-term pipeline can hurt us,” she says. On the other hand, industry has resources the botany professors can only dream of: A few days after the FFAR event, Monsanto's Schaecher made a presentation at the American Phytopathological Society meeting, a yearly powwow of more than 1,500 plant pathologists. When he pulled up the map of the BioAg Alliance's field trials, Linda Kinkel, a plant pathologist at the University of Minnesota, nearly fell out of her chair. “If they're truly collecting data on 500,000 soil microbiomes, that's more than all the rest of us put together,” Kinkel says. And she worries, “How much science is in there that we'll never see?”
Leveraging the phytobiome to improve crops will require a thorough integration of information from different sources and disciplines, but many of the parties involved are not forthcoming. Farmers are hesitant to give others access to the data they gather in their fields, citing privacy concerns. The BioAg Alliance occasionally feeds bits and pieces to academic collaborators, but Schaecher says it cannot share all its data because Monsanto and Novozymes have to “protect their competitive advantage.” In 2017 the alliance launched its first product, a microbial seed coating based on a fungus found in cornfield soil that increased yields by an average of three bushels per acre in field tests. Projections suggest the product could be used to boost crop yields on up to 90 million acres of farmland globally.